1. Introduction
2. Materials and Methods
Plant material, extraction, and isolation
Cell culture and differentiation
Oil-Red O staining of the lipid droplets
Reverse transcription-polymerase chain reaction analysis
Western blotting
Cellular alkaline phosphatase (ALP) activity
Alizarin Red Staining
Statistical analysis
3. Results
Effect of LHE on differentiation of pre-adipocytes and pre-osteoblasts
Effect of LHE on the differentiation of D1 bone marrow mesenchymal stromal cells
4. Discussion
1. Introduction
Osteoporosis is a common medical condition where imbalanced bone formation is observed. Also, studies showed that under osteoporotic conditions, bone marrow cells tend to differentiate into adipocytes rather than osteoblasts which further progress fragile bone structure (Justesen et al. 2001). Up to date, osteoporosis has been treated with a wide range of treatments targeting different mechanisms of bone buildup and employing different pharmaceuticals such as bisphosphonates, parathyroid hormone, and selective estrogen receptor modulators (Watts and Diab 2010; Kraenzlin and Meier 2011; Komm and Chines 2012).
Over the years, studies have shown that the differentiation tendencies of the bone marrow mesenchymal stromal cells (MSCs) affect the composition of bone directly (Guan et al. 2012; Zhou et al. 2014). Depending on the activated pathways, MSCs can follow a path to differentiate into osteoblast or adipocyte lineage, among others while adipogenesis and osteoblastogenesis have an antagonistic relationship (James 2013). While the adipocyte differentiation increases, osteoblast differentiation is always being suppressed (Xu et al. 2016). This results in a condition called porous bone, which is a common outcome of osteoporosis. Considering the critical balance between adipogenesis and osteoporosis, inducing MSCs in favor of osteoblastogenesis to increase bone mass is a promising target for osteoporosis treatment (Veronesi et al. 2011; Xu et al. 2016).
Regulation of MSC differentiation occurs through different pathways critically intertwined. When the cells are induced to differentiate into one lineage, inducers often suppress the differentiation into others. This leads to the idea of treating osteoporosis by inhibiting adipogenesis; hence, inducing osteoblastogenesis to relieve the loss of bone tissue. Peroxisome proliferator-activated receptor γ (PPARγ) and bone morphological protein (BMP) signaling are two pathways that induce adipogenesis and osteoblastogenesis, respectively. Inducing MSCs to differentiate into adipocytes through PPARγ activation inhibits the BMP-induced osteoblastogenesis and vice versa (Lecka- Czernik 2010; Zhuang et al. 2016). Therefore, some studies focused on developing anti-osteoporotic agents that can regulate PPAR pathway (Ing and Belury 2011; Colaianni et al. 2014).
Current drugs and treatments for osteoporosis are all costly and have harmful side effects such as osteonecrosis, impaired thinking, and gastrointestinal problems (Woo et al. 2010; Otto et al. 2011; Rizzoli et al. 2012). Some safer drugs like cathepsin K and sclerostin inhibitors have been developed (Bone et al. 2009; Ominsky et al. 2011). However, most of the time, they displayed poor biocompatibility and efficiency. Thus, novel approaches and supplements that can prevent bone volume loss and restore the bone formation homeostasis are in high demand. Recently, preventive treatment has been directed to the development of active ingredients from natural sources with minor side effects and high biocompatibility for osteoporosis (Al- Anazi et al. 2011; Jia et al. 2012; An et al. 2016). Limonium tetragonum is an edible halophyte growing in salt marshes and rocky shores of Korea and known to possess beneficial health properties including but not limited to antioxidative, hepatoprotective, and antitumor activities (Lee et al. 2011; Yang et al. 2014). Therefore, the present study investigated the potential effect of L. tetragonum on the differentiation of pre- adipocytes and pre-osteoblasts, along with bone marrow MSCs.
2. Materials and Methods
Plant material, extraction, and isolation
Whole plants of L. tetragonum were kindly supplied by Korea Maritime and Ocean University (Yeongdo, Busan, Korea). L. tetragonum was extracted with procedures previously described (Bae et al. 2016) to obtain L. tetragonum extract (LHE).
Cell culture and differentiation
Murine osteoblast-like MC3T3-E1 cells, obtained from ATCC (CRL-2593; Manassas, VA, USA), were cultured in 6-well plates unless otherwise noted and fed with α- Modified minimal essential medium (Gibco-BRL, Gaithersburg, MD, USA) containing 10% fetal bovine serum (heat-inactivated, v/v) (Gibco-BRL), 1 mM sodium pyruvate, 100 units/L penicillin and 100 mg/L streptomycin in an atmosphere of 5% CO2 at 37°C. Following the confluence, osteoblast differentiation was induced with a differentiation cocktail of 50 μg/ml ascorbic acid and 10 mM β-glycerophosphate in culture medium. LHE was introduced to the differentiating cells along differentiation cocktail, and cells were incubated for 3 days. Following medium changes did not contain LHE. Mouse 3T3-L1 preadipocytes were cultured in DMEM (Gibco-BRL) supplemented with 10% fetal bovine serum (FBS) at 37°C atmosphere supplemented with 5% CO2. On the second day after the cells reached confluence, adipogenic differentiation was induced with an adipogenesis cocktail containing insulin (5 μg/ml), dexamethasone (0.25 μM), and 3-isobutyl-1-methylxanthine (0.5 mM) in DMEM. Three days after the differentiation induction, the adipogenesis cocktail was replaced with DMEM with 10% FBS and insulin (5 μg/ml). Following a 48 hr incubation, after the confirmation of adipogenesis through cell morphology, cells were fed 10% FBS-supplemented DMEM until the time of experiments. LHE was introduced to preadipocytes with differentiation cocktail only. Murine D1 bone marrow mesenchymal stem cells were cultured in DMEM supplemented with 10% fetal bovine serum (FBS) at 37°C atmosphere supplemented with 5% CO2. Induction of adipogenesis and osteoblastogenesis were performed with the same procedures used for 3T3-L1 preadipocytes and MC3T3-E1 preosteoblasts, respectively. All reagents used for cell culture and maintenance were purchased from Sigma-Aldrich (St. Louis, MO, USA) unless otherwise noted.
Oil-Red O staining of the lipid droplets
Cell culture medium was removed at day 8 of adipogenesis, and cells were washed twice with phosphate buffer saline (PBS). Cells were then fixed on wells with 3.7% fresh formaldehyde dissolved in PBS and left at room temperature for 1 hr. Removal of fixation solution from wells was followed by the administration of filtered staining solution containing Oil-Red O (0.5% w/v) (O1391; Sigma- Aldrich). After 1 hr of incubation, the staining solution was aspirated from the plates. Images of cells were taken with a Nikon Instruments microscope (Tokyo, Japan). Oil-Red O stain was eluted from wells with 100% isopropanol and quantified by measuring absorbance value at 500 nm using a microplate reader (Tecan Austria GmbH, Austria).
Reverse transcription-polymerase chain reaction analysis
Total RNA was obtained from differentiated osteoblasts at day 4 of treatment, and from adipocytes at day 8 of differentiation using Trizol reagent (Invitrogen, CA, USA). Synthesis of cDNA was started with the addition of total RNA (2 μg) to RNase-free water containing oligo (dT) and followed by denaturation at 70°C for 5 min. Next, the mixture was reverse transcribed in a master mix (1X RT buffer, 1mM dNTPs, 500 ng oligo (dT), 140 U M-MLV reserve transcriptase and 40 U RNase inhibitor) using an automatic T100 Thermal Cycler (Bio-Rad, England, UK) with a cycle of 42°C for 60 min and 72°C for 5 min. Sense and antisense primers, which were previously detailed (Karadeniz et al. 2014), were used for the amplification of the target cDNA. The cDNA amplification was carried out using T100 Thermal Cycler (Bio-Rad) with the following settings: 30 cycles of 95°C for 45 sec, 60°C for 1 min and 72°C for 45 sec. Final PCR products were separated on 1.5% agarose gel for 30 min at 100 V. Bands were then observed following the staining with 1 mg/ml ethidium bromide under UV light using CAS-400SM Davinch-Chemi imager (Seoul, Korea).
Western blotting
Protein immunoblotting was performed with standard Western blotting procedures. Cells were harvested at the designated times given in the previous section and lysed by pipetting with 1 ml of RIPA lysis buffer (Sigma–Aldrich) at 4°C for 30 min. The cell lysate (25 μg) was used for Western blot analysis. Proteins in cell lysate were separated by sodium dodecyl sulfate-polyacrylamide gel electrophoresis (SDS-PAGE) on 4% stacking and 12% separating gels. Proteins were then electrotransferred to a polyvinylidene fluoride membrane (Amersham Pharmacia Biosciences., England, UK), which was blocked with 5% skim milk powder in TBST buffer after transfer. The membrane was incubated with primary antibodies diluted (1:1000) in primary antibody dilution buffer containing 1X TBST with 5% bovine serum albumin at 4°C overnight. The next day membranes were incubated with horseradish- peroxidase-conjugated secondary antibody at room temperature for 2 h. Immunoreactive protein bands were visualized by a luminol-based chemiluminescence assay kit (Amersham Pharmacia Biosciences) according to the manufacturer’s manual. Images of protein bands were captured using a Davinch-Chemi imager (CAS-400SM, Seoul, Korea). The primary antibodies were purchased from Cell Signaling Technology, Inc. [Danvers, MA, USA: PPARγ, #2443; CCAAT/enhancer-binding protein (C/EBP) α, #2295], Abcam [Cambridge, UK: sterol regulatory element-binding protein-1c (SREBP-1c), ab3259], and Santa Cruz Biotechnology (Dallas, TX, USA: β-actin, sc-47778).
Cellular alkaline phosphatase (ALP) activity
ALP activity was evaluated in differentiated MC3T3-E1 and D1 osteoblasts treated with or without LHE. At day 3 of LHE treatment, cells were washed with PBS and lysed with 0.1% Triton X-100 and 25 mM carbonate buffer. The cellular ALP activity was assessed using the supernatants of the cell lysates following the centrifugation at 4°C 12,000 × g for 15 min. The absorbance of the reactive solution containing the supernatants and the enzyme reaction buffer (15 mM ρ-nitrophenyl phosphate, 1.5 mM MgCl2 and 200 mM carbonate buffer, pH 9.5) was measured at 405 nm after 15 min using a microplate reader (Tecan Austria GmbH, Grodig, Austria).
Alizarin Red Staining
Calcification of differentiated MC3T3-E1 and D1 osteoblasts was quantified by Alizarin Red staining. Following three days of treatment, cells were fixed for 30 min in 6-well plates with 70% ice-cold ethanol, which was followed by the removal of ethanol and washing with distilled H2O. Staining was carried out by the introduction of Alizarin Red S (A5533; Sigma-Aldrich) staining solution followed by 10 min incubation at room temperature. After the incubation, the alizarin red solution was aspirated from wells, and cells were washed with distilled H2O to remove unbound stain. Stained cell images showing calcification were taken by an Olympus microscope (Tokyo, Japan). Subsequently, the Alizarin red dye was eluted from wells with 10% cetylpyridinium chloride (Sigma-Aldrich) solution, and calcification was quantified by absorbance values at 560 nm using a microplate reader (Tecan Austria GmbH, Grodig, Austria).
Statistical analysis
The data were presented as the mean of three independent experiments±SD. Statistically significant differences among the means of the individual test groups were determined by one-way analysis of variance (ANOVA) followed by Duncan’s post-hoc test using SAS software (version 9.1; SAS Institute, Cary, NC, USA), p < 0.05 being the defining level for the significance of differences.
3. Results
Effect of LHE on differentiation of pre-adipocytes and pre-osteoblasts
LHE (10, 50 and 100 μg/ml) was introduced to the cell culture medium of 3T3-L1 pre-adipocytes and MC3T3-E1 pre-osteoblasts with the induction of differentiation. Effect of the LHE on the differentiation of pre-adipocytes was screened by its ability to lower the lipid accumulation and suppress adipogenesis marker proteins, PPARγ, SREBP1c and C/EBPα. Non-cytotoxic presence of the LHE on 3T3-L1 cells was confirmed by the cell viability assay (Fig. 1A). Oil Red O staining of the differentiated adipocytes showed that the accumulated lipid amount was dose-dependently decreased after LHE treatment (Fig. 1B). The effect of LHE on the PPAR pathway was evaluated by the mRNA and protein levels of transcription factors. Treatment with LHE notably reduced the mRNA expression levels of PPARγ and C/EBPα, dose-dependently (Fig. 1C). SREBP1c was inhibited slightly, but no significant dose dependency was observed. Unlike mRNA expression, protein levels of all transcription factors were dose-dependently lowered by LHE treatment (Fig. 1D).
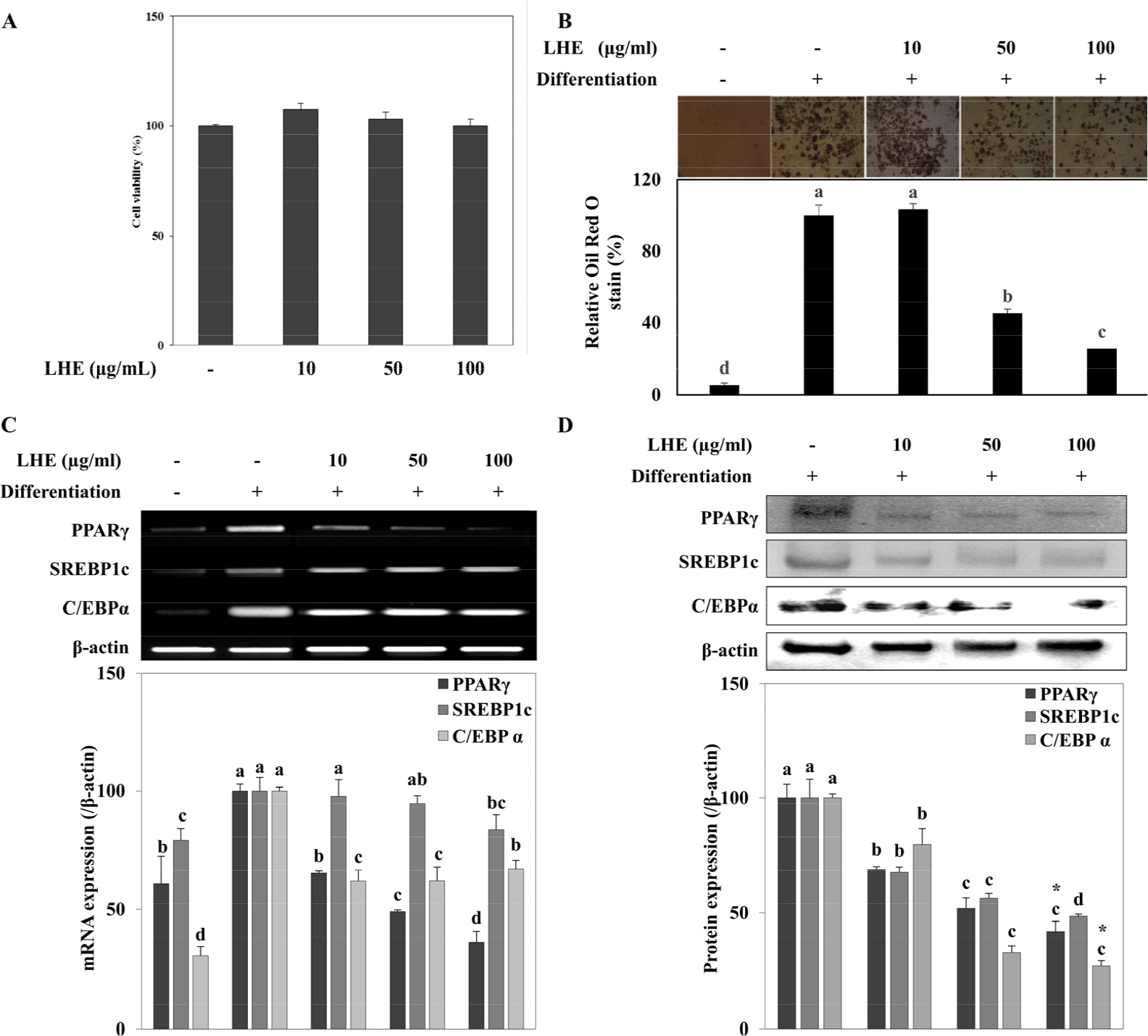
Fig. 1.
Effects of L. tetragonum extract (LHE) on; (A) cell viability of 3T3-L1 preosteoblasts, (B) lipid accumulation, (C) mRNA expression, and (D) protein levels of PPARγ, SREBP1c and C/EBPα in differentiating 3T3-L1 adipocytes. The mRNA and protein levels were quantified by band density normalized against β-actin as the internal control and given as percentage of differentiated untreated control cells. Values are means±SD (n = 3). a-dMeans with the different letters are significantly different (p < 0.05) by Duncan’s multiple range test
As for the osteogenic differentiation, the effect of LHE on osteoblastogenesis was evaluated in MC3T3-E1 cells via analyzing cellular ALP activity and ALP mRNA expression levels. Osteoblasts that were treated with 50 µg/ml LHE showed a 23% increased ALP activity compared to the untreated osteoblasts (Fig. 2A). On the other hand, 10 µg/ml treatment did not exert any notable increase, while 50 and 100 µg/ml treatment elevated the ALP activity in a similar way. Untreated osteoblasts were observed to express 95.21% more ALP mRNA compared to non-differentiated pre-osteoblasts (Fig. 2B). Interestingly, 10 and 50 µg/ml LHE suppressed the ALP mRNA expression. However, 100 µg/ml LHE treatment raised the ALP mRNA levels to 122.54% of the non-differentiated cells. To support the enhancing effect of high dose LHE on osteoblastogenesis other osteogenic markers (BMP2 and osteocalcin) were observed (Fig. 3). Expectedly, 100 µg/ml treatment raised the BMP2 levels by 17.50% compared with the untreated osteoblasts. In terms of osteocalcin, although elevated mRNA levels were observed, there was not any dose- dependency.
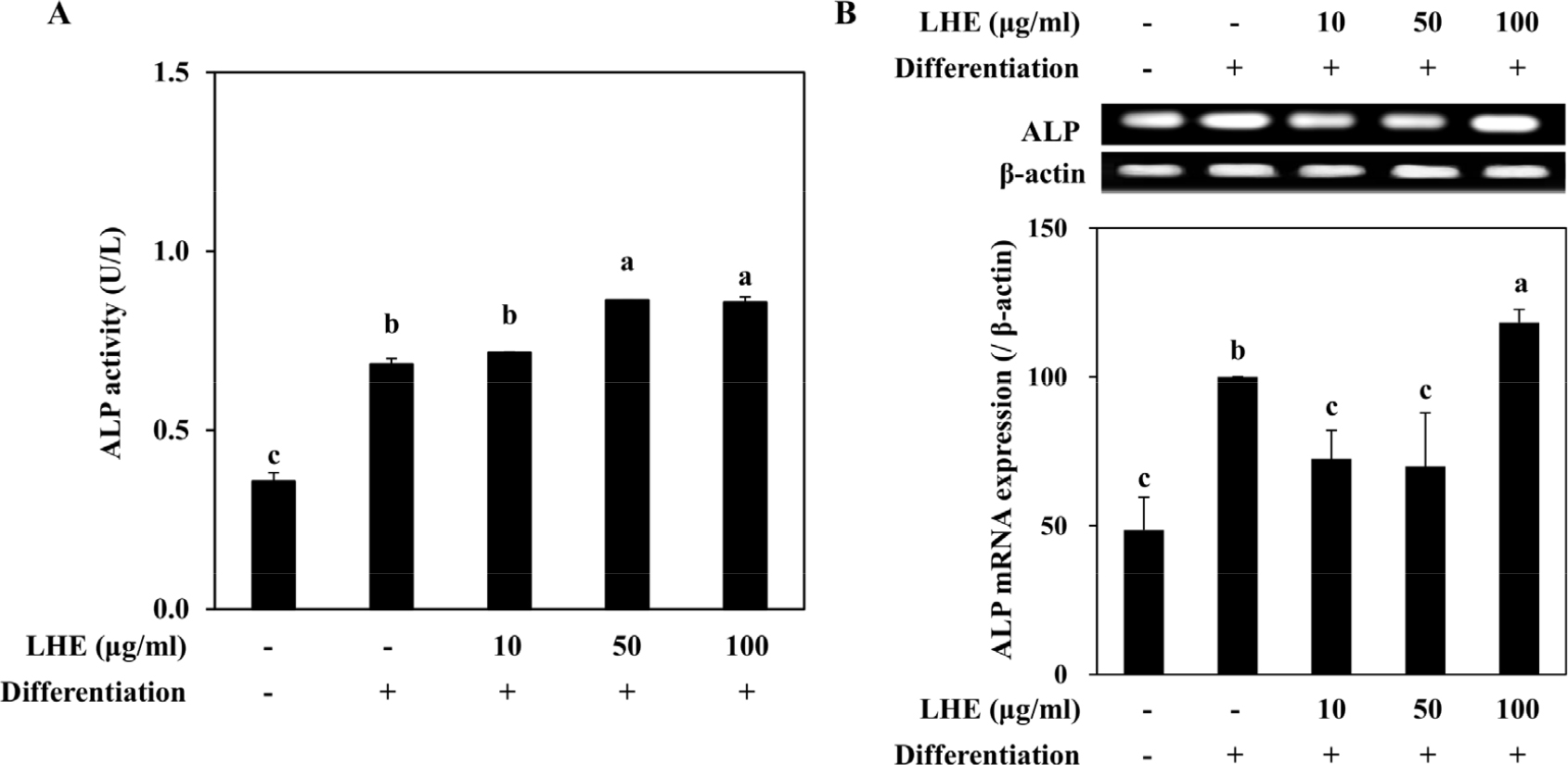
Fig. 2.
Effect of L. tetragonum extract (LHE) on the activity (A) and mRNA levels (B) of ALP in differentiating MC3T3-E1 osteoblasts. The mRNA levels were quantified by band density normalized against β-actin as the internal control and given as percentage of differentiated untreated control cells. Values are means±SD (n = 3). a-cMeans with the different letters are significantly different (p < 0.05) by Duncan’s multiple range test
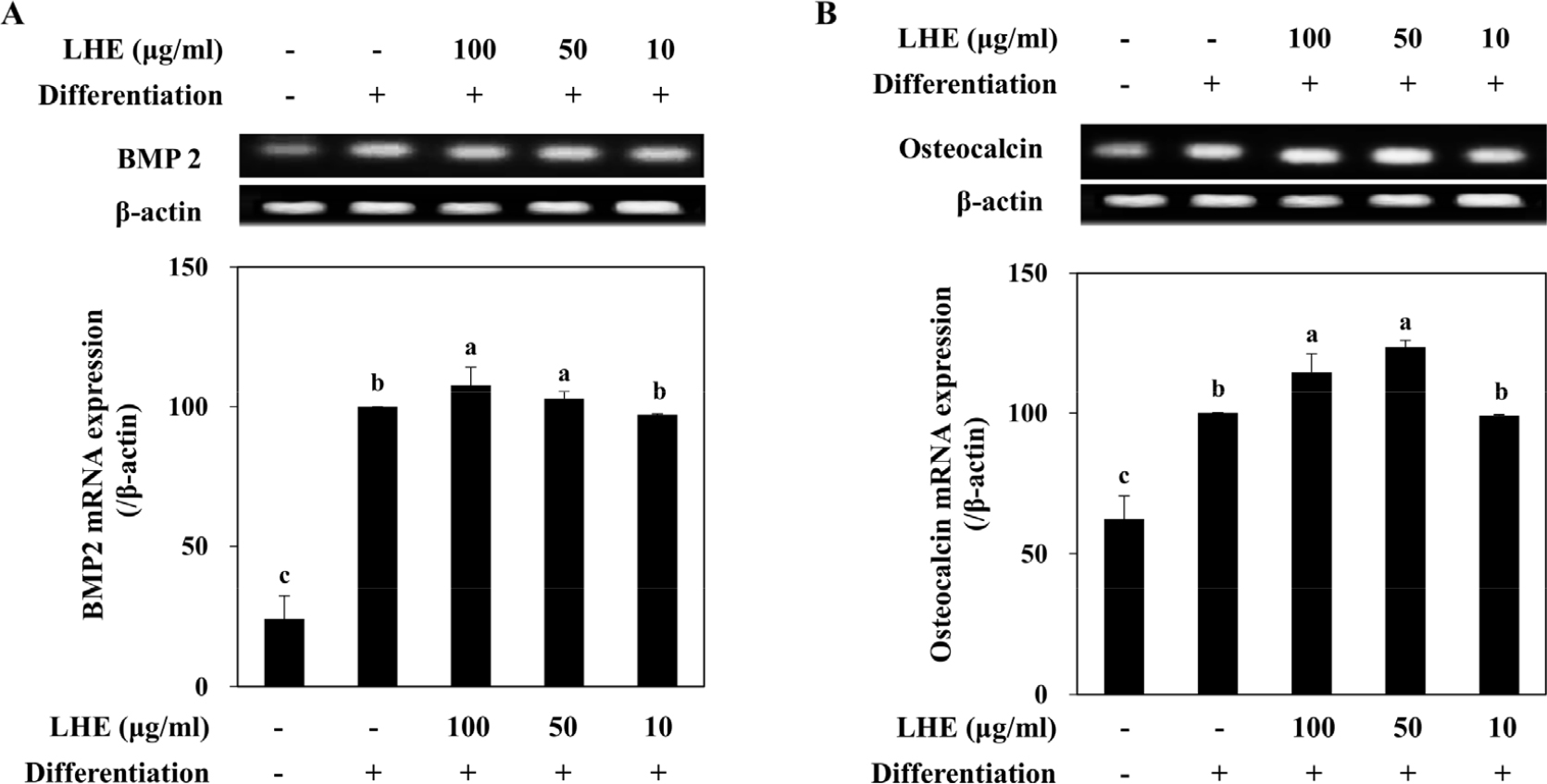
Fig. 3.
Effect of L. tetragonum extract (LHE) on the mRNA levels of BMP2 and osteocalcin. The mRNA levels were quantified by band density normalized against β-actin as the internal control and given as percentage of differentiated untreated control cells. Values are means±SD (n = 3). a-cMeans with the different letters are significantly different (p < 0.05) by Duncan’s multiple range test
Effect of LHE on the differentiation of D1 bone marrow mesenchymal stromal cells
To further evaluate the pro-osteoblastogenic and anti- adipogenic potential of LHE shown in fibroblast pre- cursors, its effects on D1 bone marrow mesenchymal stromal cells were assessed. Cell viability assay results showed that the LHE is not cytotoxic to D1 cells at the treated concentrations (Fig. 4A). Accumulation of lipid droplets in adipo-induced D1 cells was dose-dependently lowered by LHE treatment (Fig. 4B). Compared with non-differentiated D1 MSCs, mature D1 adipocytes contained 160% more triglycerides as a marker of successful adipogenic differentiation. At the highest concentration treated (100 µg/ml) LHE decreased the intracellular triglyceride amount to 53% of the non-differentiated MSCs.
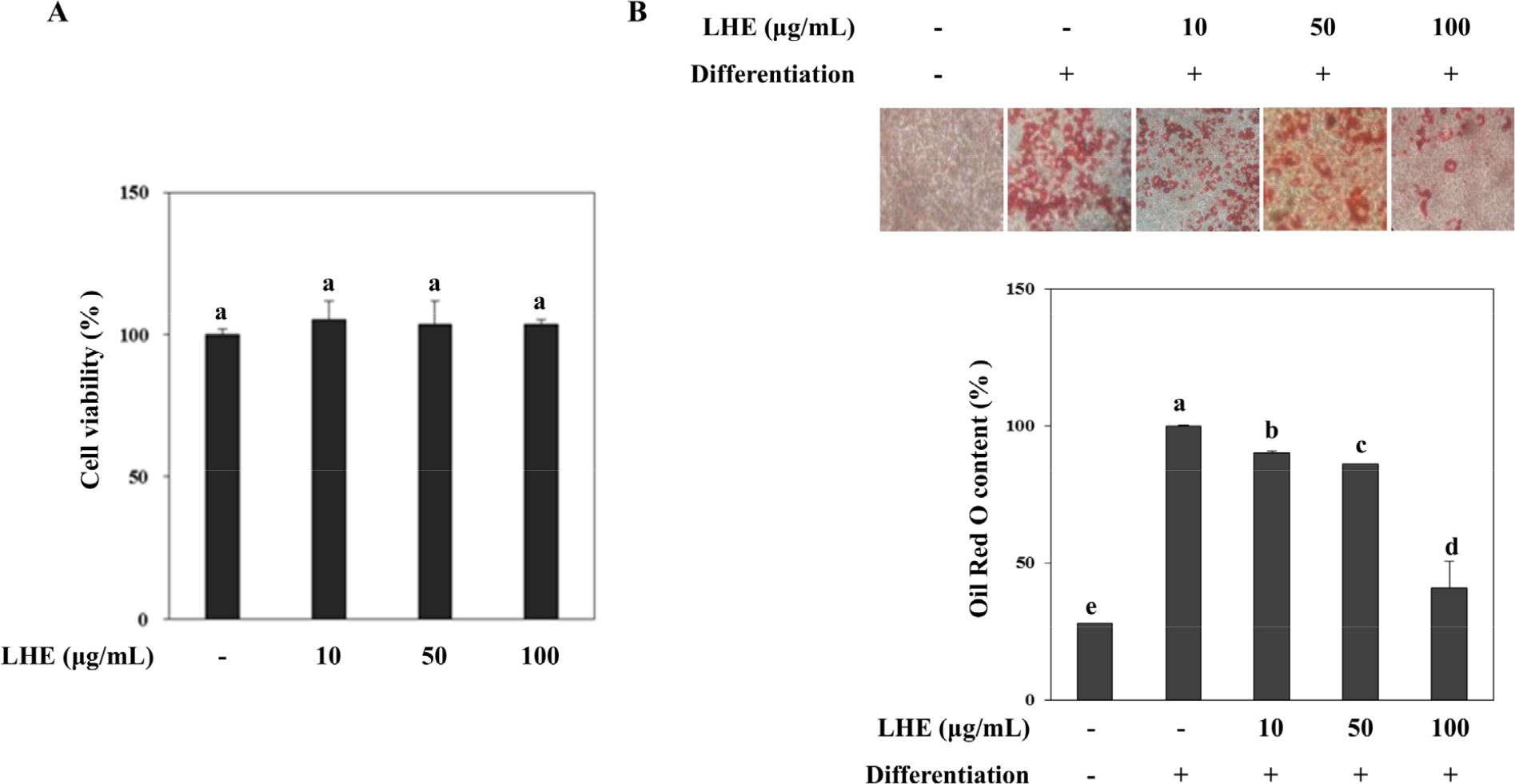
Fig. 4.
Effect of L. tetragonum extract (LHE) on the cell viability (A) of D1 bone marrow MSCs and lipid accumulation (B) in differentiating D1 adipocytes. Lipid accumulation was calculated by the quantification of the eluted Oil Red O stain bound to intracellular triglycerides and given as the percentage of the differentiated untreated control cells. Values are means±SD (n = 3). a-eMeans with the different letters are significantly different (p < 0.05) by Duncan’s multiple range test
Inhibitory effect of LHE against MSC adipogenesis was further confirmed by analyzing PPARγ pathway transcription factors. Similar to the results obtained from pre-adipocyte differentiation, LHE treatment suppressed mRNA and protein levels of PPARγ, SREBP1c and C/EBPα in D1 adipocytes at the concentrations 50 and 100 µg/ml (Fig. 5). Next, the effect of LHE on D1 osteoblastogenic differentiation was evaluated. D1 cells were osteo-induced, and ALP activity and calcification were tested as osteoblast maturation indicators. Cells treated with LHE exhibited enhanced ALP activity (Fig. 6A) and higher calcification compared to D1 osteoblasts without any treatment (Fig. 6B). D1 osteoblasts displayed 83.15% higher mineralization compared to non- differentiated cells. The increase in mineralization was 130.95% for 100 µg/ml LHE treated osteoblasts.
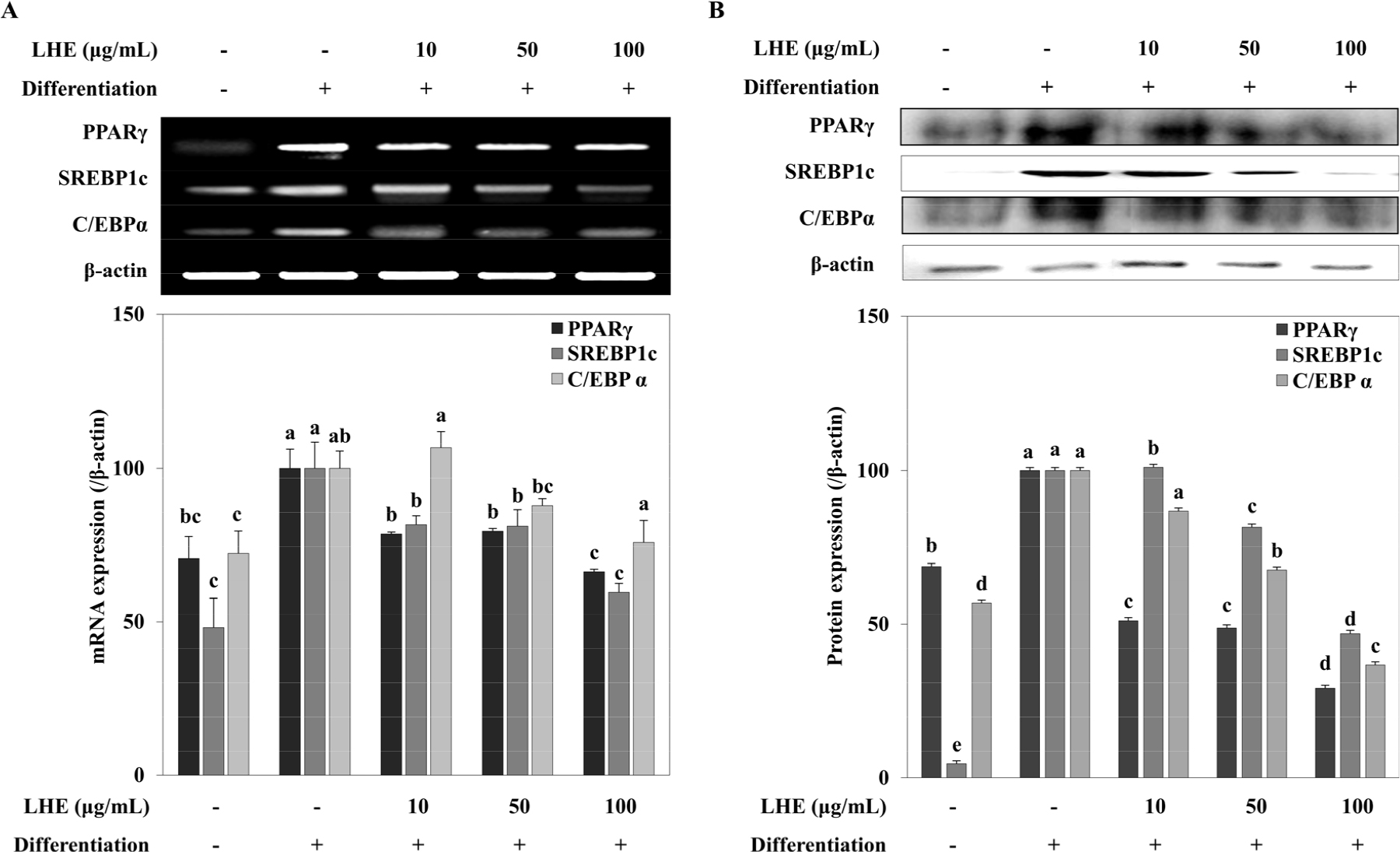
Fig. 5.
Effect of L. tetragonum extract (LHE) on the mRNA expression (A) and protein levels (B) of PPARγ, SREBP1c and C/EBPα in differentiating D1 adipocytes. The mRNA and protein levels were quantified by band density normalized against β-actin as the internal control and given as percentage of differentiated untreated control cells. Values are means±SD (n = 3). a-cMeans with the different letters are significantly different (p < 0.05) by Duncan’s multiple range test
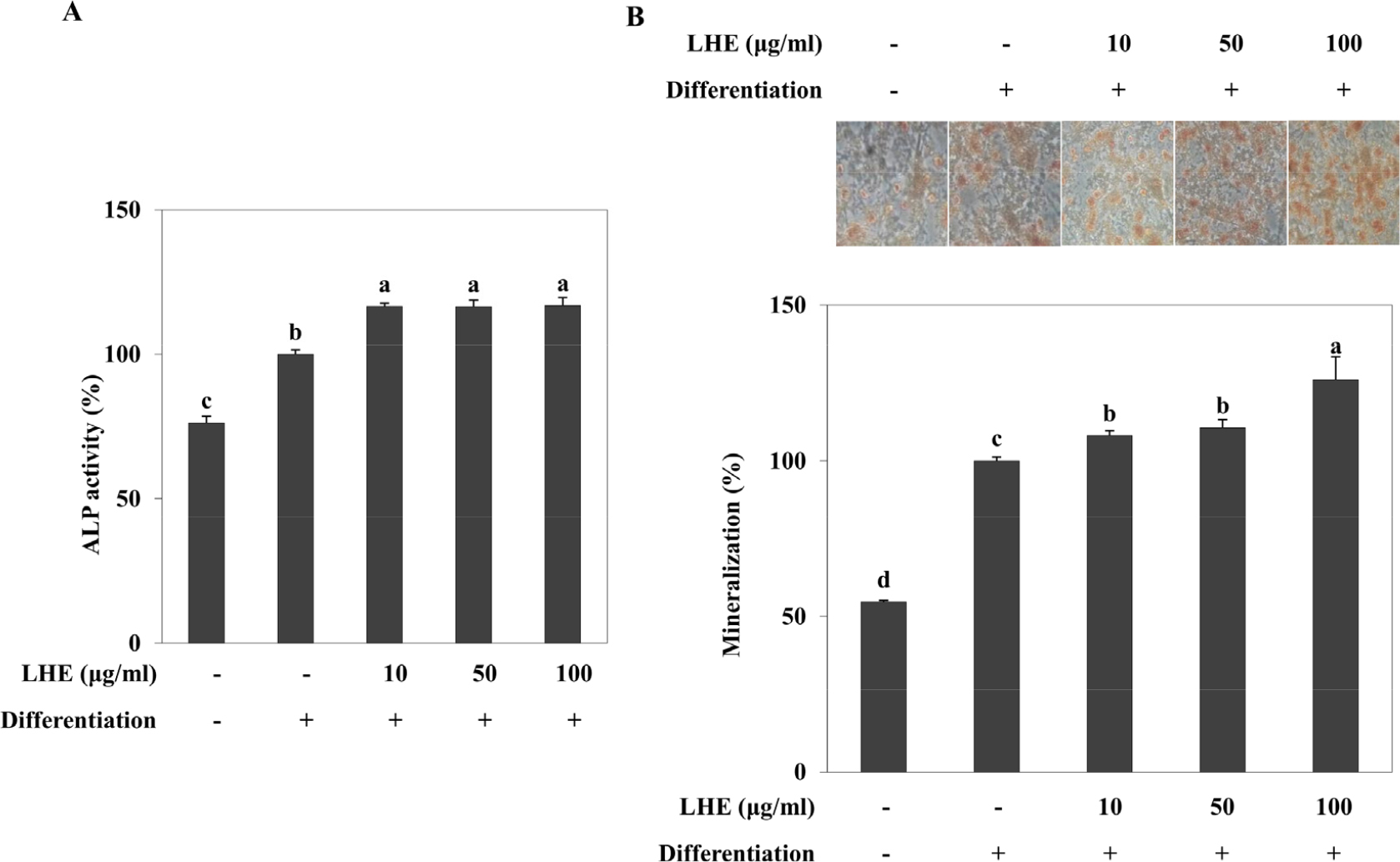
Fig. 6.
Effect of L. tetragonum extract (LHE) on the ALP activity (A) and intracellular mineralization (B) in differentiating D1 osteoblasts. Intracellular mineralization was quantified by the amount of eluted Alizarin Red stain bound to intracellular calcification in D1 osteoblasts and given as the percentage of differentiated and untreated control cells. Values are means±SD (n = 3). a-dMeans with the different letters are significantly different (p < 0.05) by Duncan’s multiple range test
4. Discussion
To determine the potential of L. tetragonum to alleviate osteoporotic conditions, its ability to enhance bone formation through osteoblastogenesis while hindering bone tissue adiposity was evaluated. Formation of bone tissue mainly carried out by the differentiation of MSCs into osteoblasts and from osteoblasts to osteocytes (James 2013). However, studies showed that under osteoporotic conditions, MSCs tend to differentiate into adipocytes resulting in decreased bone formation and excessive adipose tissue in bone (Hu et al. 2018). Therefore, targeting the imbalance between adipogenic and osteogenic differentiation of MSCs is a promising approach to treat or prevent osteoporosis-linked bone alterations (Wang et al. 2016).
Before confirming the effect of LHE on D1 MSCs, its ability to inhibit adipogenesis was tested on 3T3-L1 murine pre-adipocytes. Accumulation of intracellular lipid is characteristic of adipocytes. Moreover, the mechanism behind the adipocyte formation is regulated mainly by the PPARγ pathway, which is also involved in the suppression of osteoblastogenesis (Wang et al. 2016). LHE decreased triglycerides in mature 3T3-L1 adipocytes (Fig. 1A) and suppressed the transcription factors SREBP1c, C/EBPα, and PPARγ at both mRNA and protein levels, underlying the suggested mechanism of anti-adipogenic effect of LHE. Studies reported that excessive PPARγ activation, which is achieved by a signaling cascade including C/EBPα and SREBP1c, induces adipogenesis and fat accumulation while resulting in bone loss (Wan et al. 2007; Kawai and Rosen 2010). Therefore, after observing the LHE-mediated suppression of PPARγ pathway, the effect of LHE on osteoblastogenesis was analyzed in MC3T3-E1 pre- osteoblasts. MC3T3-E1 mouse pre-osteoblasts are currently regarded as useful in vitro models to study osteogenic differentiation. During the osteogenic differentiation, ALP is increasingly activated and expressed due to its role in the calcification of the newly formed bone tissue (Golub and Boesze-Battaglia 2007). The LHE treatment of osteo- induced MC3T3-E1 pre-osteoblasts at the concentration of 100 μg/ml resulted in elevated ALP activity along with increased ALP mRNA expression (Fig. 2). However, at lower doses (10 and 50 μg/ml) it was observed that LHE treatment suppressed the differentiation-dependent increase in ALP mRNA expression. Previous reports suggested that the ALP activity and expression during osteogenic differentiation are induced by BMP2 (Matsubara et al. 2008). Over the years, anabolic therapies targeting BMP2 and BMP7 were emerged in preclinical trials due to their ability to induce bone formation (Bishop and Einhorn 2007). In 2002, BMP2 was approved by FDA for spinal fusions and open long bone fractures. In addition, the suppression of PPARγ in MSCs was shown to activate the BMP2 pathway that induces MSCs to undergo osteogenesis. In this context, the effect of LHE on the BMP2 pathway was suggested to be linked with its inhibitory effect on the PPARγ. Results were in accordance, as LHE treatment at the concentration of 100 μg/ml resulted in slightly enhanced BMP2 mRNA expression in osteoblasts (Fig. 3). In addition, osteocalcin, a downstream effector of BMP pathway and linked with ALP activity, was enhanced in terms of mRNA expression.
D1 murine bone marrow MSCs was used as in vitro model for the bone formation as these cells are derived from bone marrow and able differentiate into both adipocytes and osteoblasts. Decreased amounts of lipid accumulation were observed in adipo-induced D1 MSCs after LHE treatment (Fig. 4). The levels of SREBP1c, C/EBPα, and PPARγ mRNA and protein expression after LHE treatment confirmed the suppressing effect of LHE in D1 adipocytes (Fig. 5). The PPARγ suppression by LHE would suggest stimulation of BMP2-regulated osteoblastogenesis in MSCs similar to its effect of pre- osteoblasts. According to Alizarin Red S staining results, extracellular calcification during the D1 osteoblastogenesis was further stimulated by LHE treatment (Fig. 6).
Overall, the effect of LHE on both bone marrow MSCs and pre-adipocytes and pre-osteoblasts suggested that LHE might contain bioactive substances that can hinder bone marrow adiposity while stimulate the MSC differentiation to osteoblasts. This regulatory effect might enable LHE- derived bioactive compounds to be utilized as anti- osteoporotic nutraceuticals. However, future studies on LHE are needed for the elucidation of bioactive substances from the extract and evaluation of underlying mechanisms using human in vitro models. Nonetheless, LHE possesses a pro-osteogenic and anti-adipogenic properties. These health benefits may promote the use of L. tetragonum as a source of bioactive ingredients useful in bone-related disorders.